The paper was published in Nanoscale (2022).
The surface has always been of particular interest because of its wide variability in structure and unexpected properties. On the other hand, “surface of surface” – an edge of a nanostructure might become important and bring new phenomena. The precise fabrication of edges on 2D materials at a certain crystallographic orientation is challenging and requires precise knowledge about edge chemistry. In some cases, edge displays very specific structure. For example, multilayered graphene edges tend to connect with each other. The case of bilayered graphene was investigated in detail and it was shown that the connection of the edges even does not require the overcoming of any barrier and, therefore, hollow sp2-hybridized graphene structure forms spontaneously. In our previous work was shown that the structure of the graphene closed edges is precisely defined and can be represented as the grain boundary between two graphene domains generally misoriented.
The understanding of the edge structure is important also for the case formation of holes in the 2D structure because this is an attractive object for tuning the material properties. For the single-layer graphene, many studies have explored holes for DNA sequencing, gas sensing, ion and molecules sieve, (in particular water desalination), molecular transport and others. Several comparable studies also have been done with hexagonal boron nitride (h-BN) and molybdenum disulfide (MoS2). The variety of hole shapes and the type of passivation of their edges does not allow systematic experimental studies to be carried out. Usually, studies focus on performance tests without the information of edge configuration and chemical stability which may affect to the performance significantly.
The carbon counterpart, boron nitride is less investigated in such a case, although it seems promising to create holes not in bigraphene, but specifically in bilayered h-BN. Indeed, the strong tendency of the layers to be AA’ stacked allows one to be confident that the bilayered structure will be predetermined. However, it remains completely unclear which edges of the bilayered h-BN will tend to close and what the final structure will be. The structure of the edges of multilayered h-BN is usually unknown whereas from general logic the similar effect of self-passivation can be expected due to the close values of flexural rigidity and edge energy.
The presented paper is devoted to the study of the edges of bilayered h-BN. It is shown that the edges tend to connect regardless of the edge cut. A defectless connection can be expected only in the case of a zigzag edge, while in other cases a series of tetragonal, and octagonal defects will be formed. This result was obtained by carrying out an analogy between the edge of bilayered h-BN and the interface of monolayer h-BN (see the Figure). Information on the structure and energetics of closed edges allowed us to predict the shape of holes in h-BN, which agreed with the reference experimental data. Finally, it is shown that the closed edges do not create states in the band gap, thus not changing the dielectricity of h-BN.
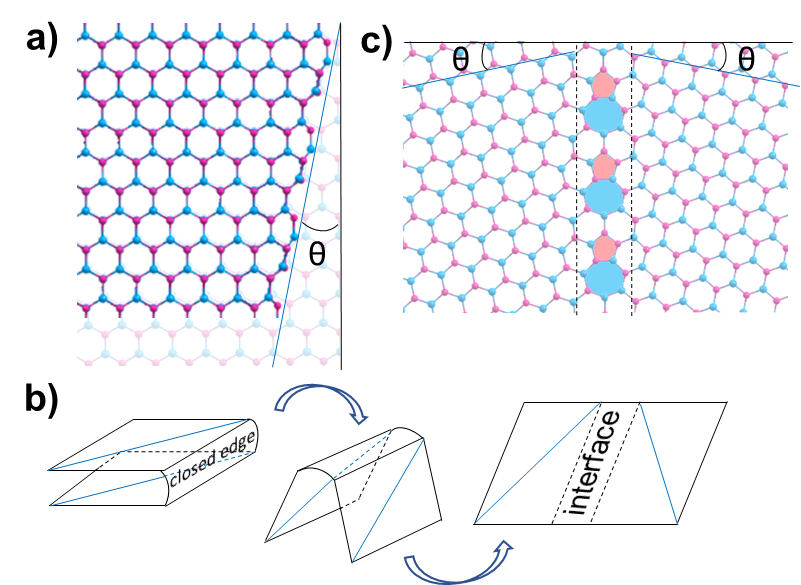
a) The top view of bilayered h-BN with closed edge. θ is the boundary inclination with respect to armchair direction. b) The scheme illustrates how a bilayered h-BN with a closed edge can be unfolded into a flat structure. c) Flat layer h-BN with a grain boundary obtained from a bilayer with a connected edge shown in (a).