Formation of diamane in bigraphene on langasite under irradiation with a electron beam
- Pavel Sorokin
- Dec 10, 2022
- 4 min read
The paper was published in Nanomaterials.
The prevailing surface contribution allows the modification of the multilayer graphene structure by surface functionalization which leads to the connection of the layers to form thinnest possible diamond film, diamane. Adsorption of reference atoms regulated by external conditions, by choosing appropriate temperatures and pressures, changes the structure of the film globally. However, this approach is not suitable when local manipulation of atomic geometry is required. It can be realized by using the biased enhanced nucleation approach allowing diamond nucleation by exposing direct electron irradiation of selected regions.
Use of electron irradiation for realizing of the chemically induced phase transition was demonstrated in our previous work where the induced formation of diamond nanocluster in the carbon network was shown. Hydrogen atoms displaced from the dodecyl groups by the “knock-on” effect penetrate the layered carbon and form a dense amorphous hydrogenated carbon (C:H) phase with the final precipitation of sp3 carbon clusters. It is attractive to extend this approach to the two-dimensional case, where an easily accessible surface allows local phase state tuning and enables the formation of a heterostructure consisting of regions with different conductivities. The latter can become the basis for nanoscale electronic devices.
The presented study is devoted to the investigation of the effect of chemically induced phase transition in bilayer graphene transferred onto a langasite substrate and irradiated with a focused electron beam through a layer of poly(methyl methacrylate). Transport measurements show that the resistance of the graphene bilayer after irradiation with an electron beam increases significantly, and the linear dependence of current on voltage in the bias voltage range from –1 to 1 V changes to a nonlinear one.
Our estimates show that the fraction of sp3 carbon in the irradiated region is about 10^12 cm–2 (see the figure below). When a certain local area of the sample is irradiated with a focused electron beam, hydrogen is released from the destroyed polymer on the one side, and oxygen from the langasite substrate on the other side. We suppose that active O and H atoms easily bind to the graphene surface which leads to the corrugation of the layer with displacing of the neighboring atom from the plane owing to sp3 hybridization. Such behavior of graphene is typical upon attachment of reference atoms to it. In the case of a single layer of graphene in this way, it is possible to obtain the “ultimate diamond slab” (graphane or fluorographene for the cases of H and F adsorption, respectively). The deposition of a reference atom to the carbon leads to the rehybridization of its bonding from sp2 to sp3, leading to a change in the chemistry of neighboring C atoms, that in turn tend to connect with other atoms. In the case of a multilayered film, such atoms are carbon atoms from the neighboring layer which leads to the bonding of the layers to each other and the final formation of a diamond film.

sp3 defect density distribution (cm-2) estimated from Raman D peak
In the present case, graphene is exposed to oxygen atoms from one side and hydrogen atoms on the other side forming a Janus diamane structure. We designed the corresponding model of diamane film arranged on the LGS substrate functionalized by the hydrogen atoms from the outer side. During the relaxation surface oxygen atoms of langasite shifted and connected with the carbon atoms on the interface stabilizing diamane geometry. The final structure of hexagonal diamane film with the surface (see figure (a) given below) showed high stability which proves the experimental suggestion of bilayer graphene diamondization by treatment by H and O-atoms. Note, that the cubic diamane (111) structure was not stabilized by oxygen and was partially graphitized.
The observed high resistivity of the diamondized graphene regions is explained by the density of electronic states (see figure (b) given below) where formed diamane displays a band gap of ~0.7 eV. Thus, irradiation leads to the controllable formation of regions with the large barrier in the bilayered graphene. The smaller value of the band gap (in comparison with perfect diamane which band gap is more than 3 eV) can be explained by the presence of carbon atoms with unsaturated bonds at the interface not connected with oxygen.
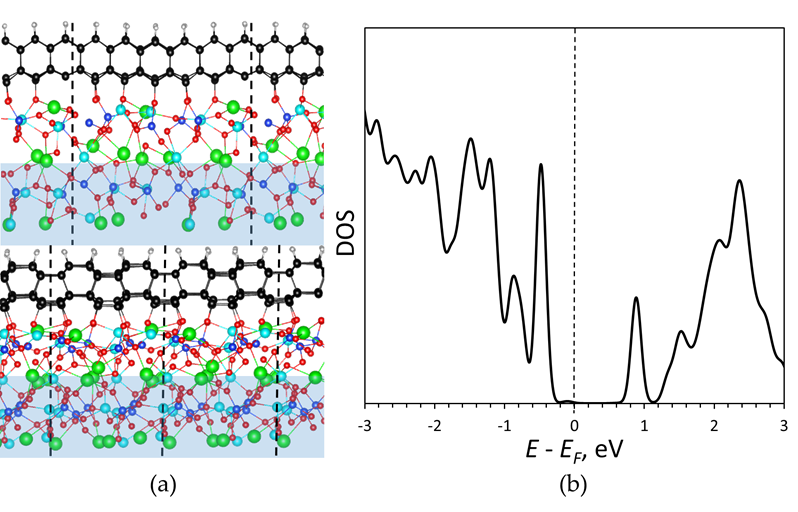
a) Atomic model of a diamane film with surface which outer side is passivated by hydrogen atoms, and the other (at the interface) is connected with oxygen atoms of the langasite substrate. Carbon, hydrogen, oxygen, lanthanum, gallium, and silicon are marked by black, gray, red, green, cyan, and blue colors. Unit cell is depicted by dashed lines. The frozen region is marked by blue; b) Partial density of electronic states of the diamane (carbon and hydrogen atoms) from the diamane/langasite structure. The Fermi level is shifted to zero
It should be noted that the low sp3 carbon density observed in modified bigraphene indicates the formation of diamane nanocluster in irradiated area. It is probably caused by the non-optimal stacking of carbon atoms in two functionalized graphene layers, which is determined by the angle of rotation between the two layers. Other possible reasons are structural and technological defects that arise during the CVD growth of graphene and the process of transferring two layers of graphene onto a substrate. As calculations shown, not all bilayer graphene stacking can be transformed into diamane, so we can assume selectivity in the connection of twisted graphene containing areas with different packages. Therefore, fine control of structural and technological defects during the formation of the bigraphene/langasite structure and its irradiation with an electron beam are suggested to obtain high-quality diamane nanostructures. As a result, an experimentally observed barrier for the carriers transfer in the bigraphene/diamane/bigraphene nanostructure appears.
Comments