The paper was published in Diamond and Related Materials.
The nanostructuring of 2D materials has attracted increasing attention recently due to its wide variety of applications, including field-effect transistors, photonic and photovoltaic devices, biosensors, analyzers of biomolecule properties, storage devices, advanced materials, and microelectromechanical systems. The creation of nanoporous layers is an important tool for the modification of 2D material properties.
In the present study, we have revealed the possibility to modify the parameters of few-layer graphene and, first of all, the size of the pores as a function of ion irradiation energy and pristine graphene structural features, and morphology. The maximum pore density is approximately equal to the ion dose found in blisters. The pore size depends on the size of the domains in graphene and ion energy. The formation of more complex structural defects in few-layer graphene is also observed after irradiation. Thus, the use of CVD graphene provides opportunities for controlling the structure and properties of the material modified by high-energy ions.
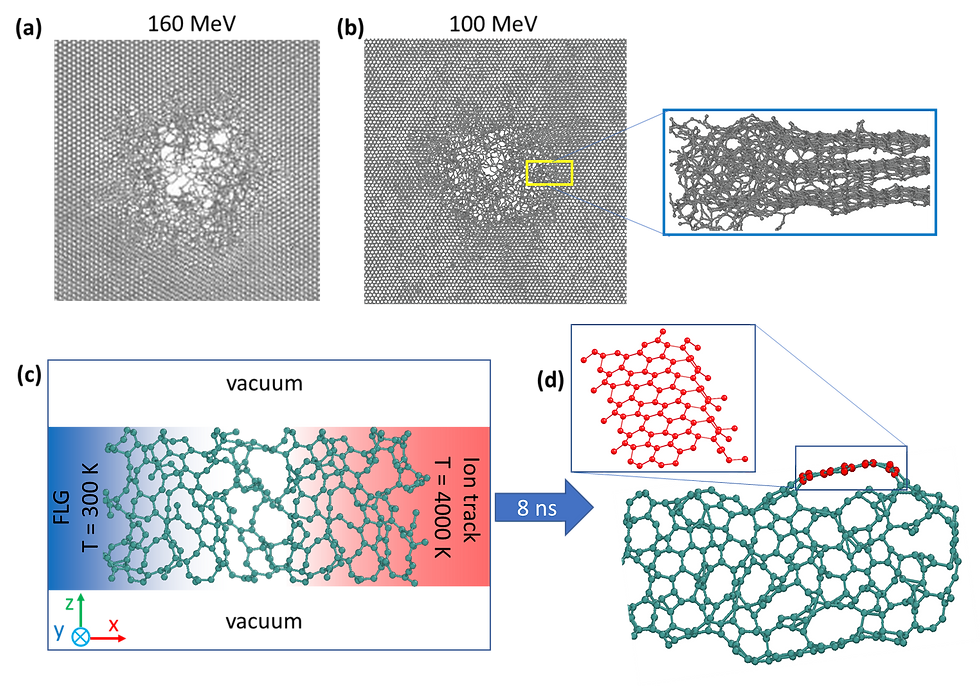
Typical graphene structure after the irradiation by Xe ion with energy a) 167 MeV and b) 100 MeV. The size of the formed pore is ~ 5×5 nm2. c,d) Behavior of the amorphized carbon ribbon, periodic only in the direction perpendicular to the figure plane (y-direction), under annealing conditions (see text for details).
We found that the decreasing of incident Xe energy from 167 to 100 MeV sufficiently change the behavior of the system. Irradiation with such energy leads to the structure amorphization with almost no evaporation of carbon from the structure. The amorphous carbon remaining in the structure can transform back into the sp2 system after cooling (healing effect), or it can change to other phase states, which are abundantly observed in the experiment.
Nevertheless, if we consider only a fragment of the edge of the multilayer graphene structure (Figure b) next to the ion track and perform long-term molecular dynamics, we can get some idea of what happens in the irradiated material already during cooling. Figure 9c shows an amorphized carbon ribbon, periodic only in the direction perpendicular to the figure plane (y-direction), with a cell parameter equal to 4.84 Å. The carbon atoms on the left edge of the ribbon are grouped and connected to the "cold" multilayer graphene thermostat at room temperature. The atoms on the right side are respectively grouped and connected to the thermostat of the material in the area of the track just after the irradiation at a temperature of 4000 K. Molecular dynamics are then performed for a few nanoseconds, during which time the temperature in the track decreases and the carbon in the middle of the ribbon is annealed and transformed into more favorable phase configurations. After a series of calculations, where the initial amorphous structure and cooling time were varied, it was observed that most of the transition of the resulting structure to sp2 hybridization of the carbon on the surface of the ribbon (Figure d). This effect can explain the stability of the outer graphene layer in the FLG irradiation experiments. The Xe ion must transfer noticeably less energy to the outer layer than to the inner layers because of the cascade effect, which is also applicable to electron stopping. The inner layers are exposed not only to the ion but also to the electrons knocked out of the previous layers, which adds up to the value of the average ion loss for ionization. The outer layer is only affected by the ion, so the energy transferred may not be enough to evaporate the carbon from the outer layer, and the amorphized structure may heal into graphene over time, as occurs in the numerical simulation. This could explain the observation of formations in few-layer graphene with the undistorted outer layer in the places of ion incidence.
Comments